Timeline: the past, present and future of sequencing technologies
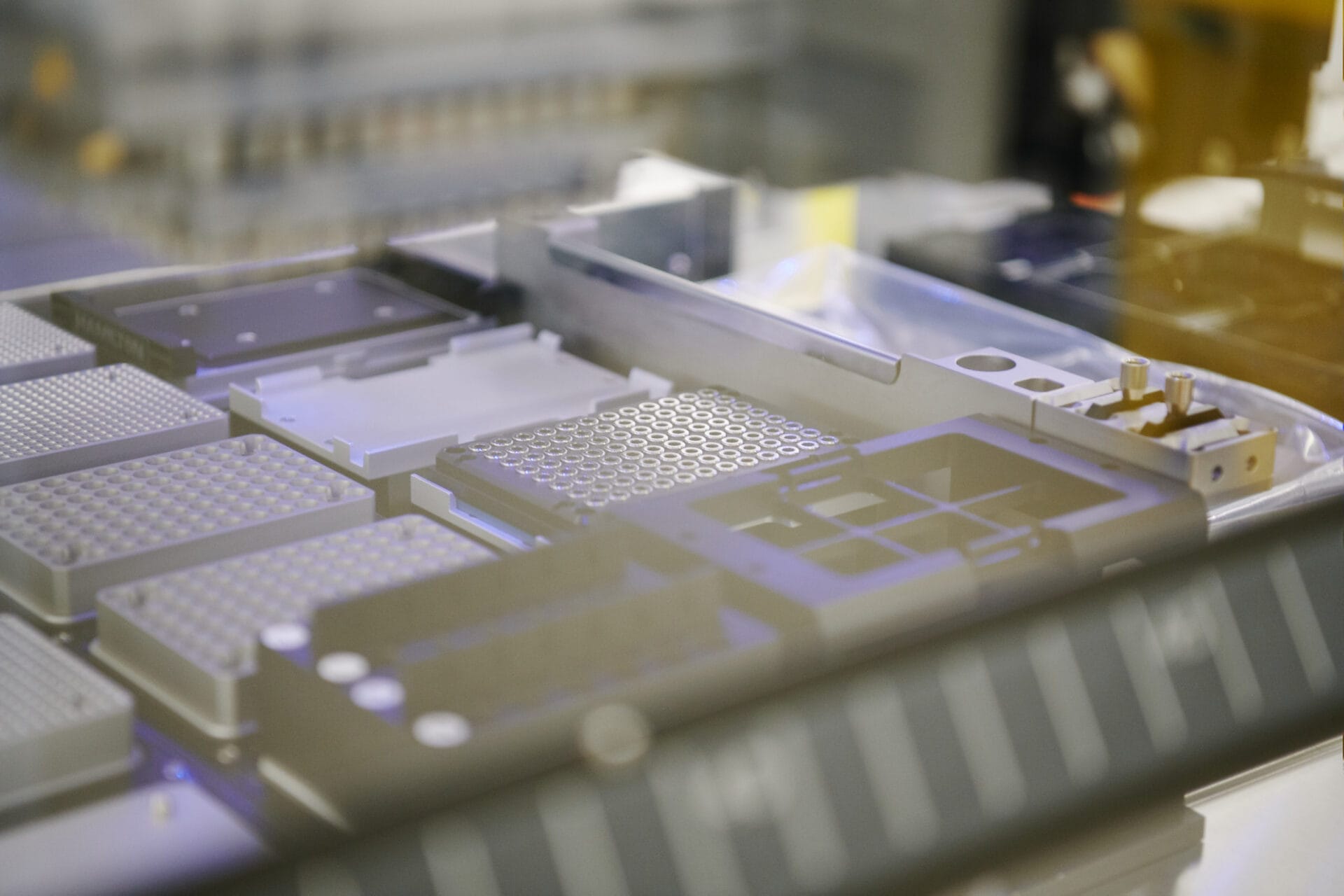
Since the 1970s, scientists’ ability to sequence a genome has evolved from a laborious, expensive process to one that is quick, accurate and able to reveal important information about biology and medicine.
Key terms
DNA
(deoxyribonucleic acid) A molecule that carries the genetic information necessary to build and maintain an organism.
DNA sequencing
The process of determining the order of bases in a section of DNA.
- In 1977, a breakthrough from Fred Sanger and his team in Cambridge, UK, made it possible to sequence a genome for the first time.
- Sequencing technologies continued to evolve in the decades that followed, dramatically reducing the speed and cost of sequencing a genome.
- This has given scientists the ability to compare the genomes of many different people and begin to uncover the genetic causes of conditions like cancer, diabetes and neurodegeneration.
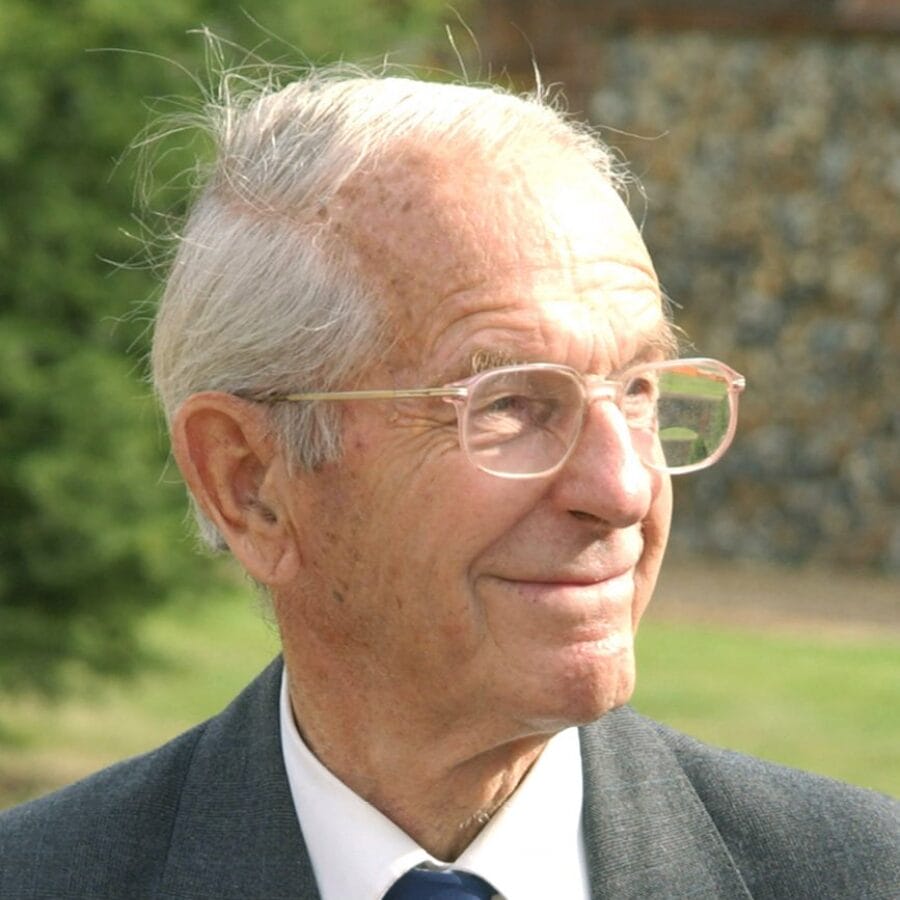
"A knowledge of sequences could contribute much to our understanding of living matter."
Fred Sanger, Nobel-laureate who pioneered DNA sequencing
The evolution of DNA sequencing technologies
The Sanger method, also known as the chain termination method
Sanger sequencing is based on natural DNA replication and results in billions of radioactively labelled DNA fragments of varying lengths. Fragments are separated on a gel and analysed by hand.
- Benefits: DNA can be sequenced for the first time
- Limitations: labour-intensive and uses radioactivity
- Cost to sequence 1 million bases: not possible
- Time to sequence a human genome: not possible
- Bonus fact: Sanger and his team were the first to sequence a whole genome using this technique, the 5,000 base-long genome of a virus called PhiX174.
Find out more about Sanger Sequencing
Automated sequencing
This automated evolution of Sanger sequencing replaces radioactive bases with coloured dyes (A = Green, C = Blue, G = Yellow and T = Red) which are read with a machine.
- Benefits: safer, automated and possible to sequence longer sequences, making it scalable
- Limitations: the gel still has to be checked by eye
- Cost to sequence 1 million bases: £10,000
- Time to sequence a human genome: 600 years
- Bonus fact: Automated sequencing machines became commercially available in the late 1980s.
Capillary sequencing (sometimes called first-generation sequencing)
Sanger sequencing evolves to use a long, thin capillary fibre rather than a gel to separate the DNA fragments. The machine makes the coloured bases fluoresce then reads and records their sequence.
- Benefits: significantly quicker and higher resolution than gel-based techniques
- Limitations: it’s not as fast or cheap as more modern techniques
- Cost to sequence 1 million bases: £3,457
- Time to sequence a human genome: 13 years
- Bonus fact: Used to sequence the first human genome, for the Human Genome Project
Next generation sequencing (or second-generation sequencing)
A further evolution of Sanger capillary sequencing that can now analyse millions of samples simultaneously. This is sometimes called ‘massively parallel sequencing’.
- Benefits: high throughput
- Limitations: can struggle with counting a string of identical bases (eg five As in AAAAA)
- Cost to sequence 1 million bases: 34p
- Time to sequence a human genome: 7-10 days
- Bonus fact: the first method to sequence a billion bases in a single day and to bring sequencing a whole genome below $600
Read more about next generation sequencing.
Third-generation sequencing
Using a completely different principle to Sanger sequencing, third generation technologies can decode much longer stretches of DNA fragments, called long reads. Techniques include PacBio’s Single-Molecule Sequencing in Real Time (SMRT) and Oxford Nanopore Technologies (ONT).
- Benefits: Single molecules can be sequenced for the first time – revealing information about how individual cells function. ONT is small and portable, making it more accessible to use.
- Limitations: its speed can lead to errors – but unlike older techniques, the errors are random, meaning they can be corrected by repeating the sequencing.
- Cost to sequence 1 million bases: from 5p – although some specific techniques are more expensive
- Time to sequence a human genome: About a day
- Bonus fact: In 2015, ONT it was used as part of an Ebola outbreak to read the genomes of Ebola viruses from 14 patients in just 48 hours.
Read more about third generation sequencing
Onwards: Future generation sequencing
Since 1977, sequencing techniques have dramatically evolved – from early techniques that enabled scientists to tap into a genome for the first time, to the direct, real-time sequencing of single DNA molecules.
Fourth generation sequencing starts to combine the benefits of the previous methods: processing large numbers of samples, quickly and accurately, with enough detail to sequence single molecules right up to whole genomes.
As technologies continue to evolve, sequencing continues to improve our ability to diagnose people with conditions more quickly and match people with genetic conditions to the right treatment for them.
1970s | 1990s | 2000s | 2010s | 2020s | |
---|---|---|---|---|---|
Generation | 1st generation with gel-based methods | 1st generation with capillary methods | Next generation | Third generation | Future generations |
Technologies | Sanger sequencing (manual) | Sanger sequencing (automated) | Illumina, Roche 454, Ion Torrent | PacBio SMRT, ONT | Genapsys, MGI |
Breakthrough | Gel-based analysis | Capillary analysis | High throughput | Long reads | |
Pros | Accuracy | Higher scale, Lower cost, Accuracy | Long reads, Ultra-fast, Portability | Aiming for cost effectiveness, accuracy | |
Cons | Labour intensive, High cost | Short reads make analysis more difficult | A reduction in accuracy |