Fruit flies in the laboratory
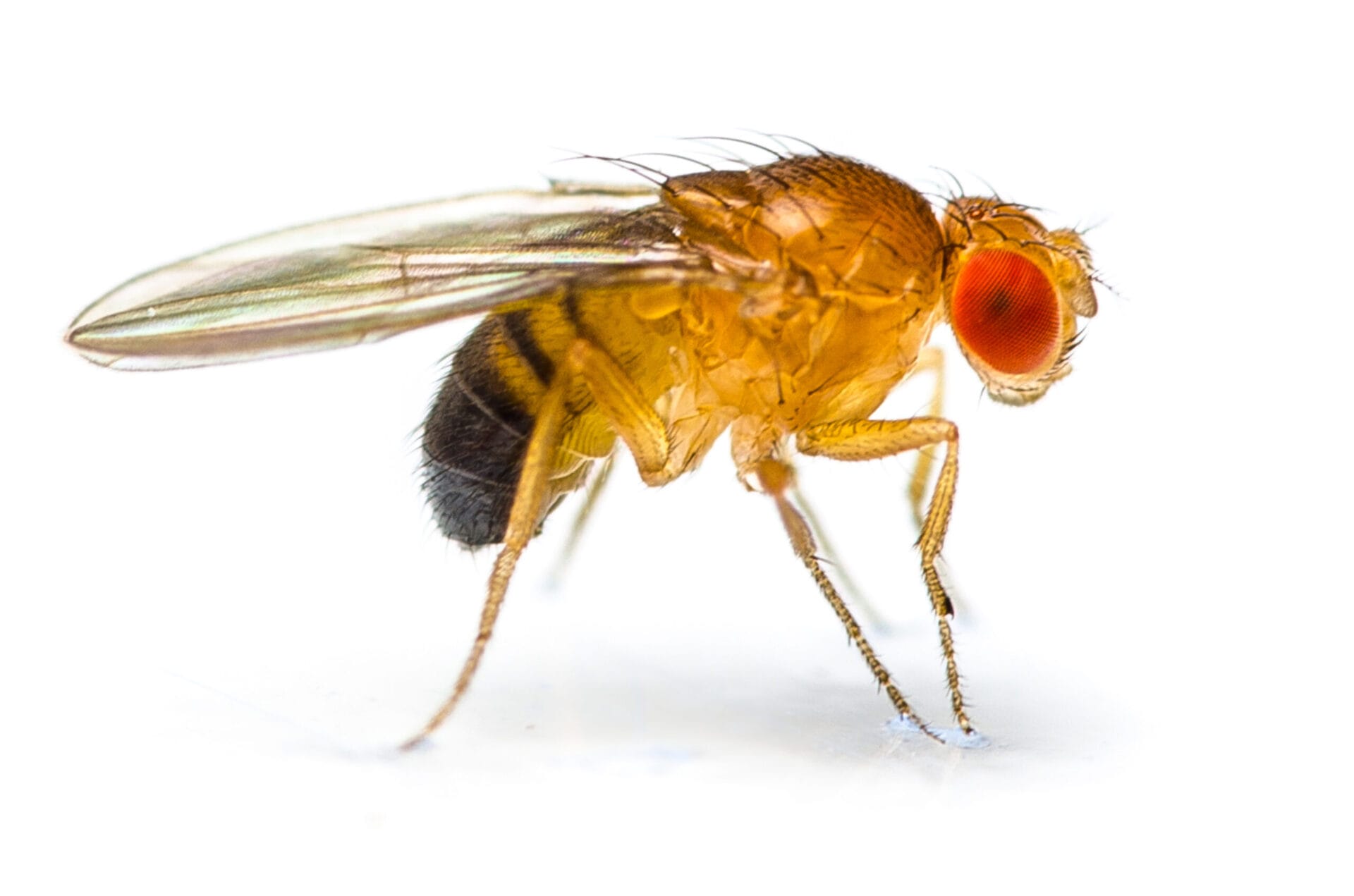
The fruit fly, or Drosophila melanogaster, has the longest history in genetics and research out of all the model organisms.
- Although generally considered a pest by farmers because they lay their eggs in ripening fruit, the fruit fly is one of the most useful tools in the laboratory.
- Many qualities make the fruit fly a useful model organism, including its short life cycle, low cost to maintain and the close relatedness of its genes to those of humans.
- The fruit fly has been used to study a whole range of biological processes, from genetics to ecology to alcoholism. Read more on our fact page.
Key terms
Model organism
A species that has been widely studied in biology, usually because it is easy to maintain and breed in a laboratory setting and has particular experimental advantages.
Gene
A section of DNA within a genome that carries a specific set of information - often the information needed to make a protein.
Mutation
A change in the DNA sequence, which may have positive, negative or neutral effects on the organism.
History of the fly
The story of the fruit fly (also known as Drosophila melanogaster) as a model organism begins in the early 1900s. Gregor Mendel’s work on inheritance had been rediscovered, but scientists still had a very limited understanding about how inheritance worked.
Embryologist Thomas Hunt Morgan started working with Drosophila in 1908. Considered the founding father of Drosophila research, and arguably the father of genetics in the US, Thomas was most interested in studying its embryology – the science of the development from the point of fertilisation to that an embryo forms.
Speculation that chromosomes might in some way be linked to an organism’s characteristics spurred Thomas’ interest in inheritance. At that time he was very sceptical of Mendel’s laws of inheritance and did not agree with Darwin’s ideas about natural selection. However, he was about to make a finding that would change his opinions completely.
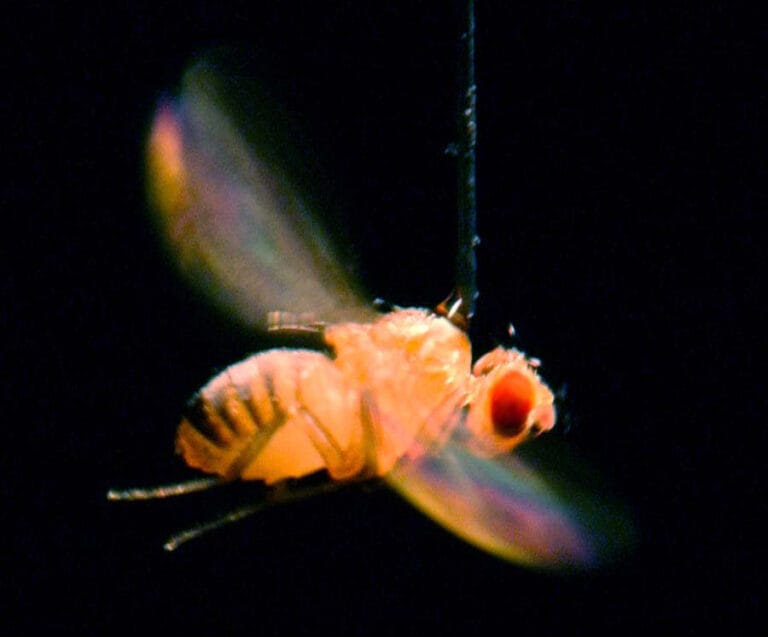
Chromosomal theory of inheritance
After a frustrating and fruitless two-year search for Drosophila with different characteristics, white-eyed flies suddenly appeared among Thomas Morgan’s normal, red-eyed flies.
To find out more about these white-eyed flies, Thomas carried cross-fertilised the white- and red-eyed flies. Through these early experiments he found that all the white-eyed flies being produced were males, there were no white-eyed females at all.
After further crosses Thomas observed that the female flies only showed the white-eyed trait if they inherited two copies of the mutant genes, while males only needed one copy of the mutant gene to have white eyes. This suggested to him that inheritance of the white-eye trait might have a basis in the chromosomes, more specifically the sex chromosomes.
At that time, little was known about the sex chromosomes, although it was thought that one of the Drosophila’s four chromosome pairs was likely to be involved in sex determination.
Thomas continued his work looking at a number of different traits in the Drosophila and in 1915 he published his theory Mechanism of Mendelian Heredity, acknowledging that he agreed with Mendel’s concept of dominant and recessive traits.
He also introduced the concept of genes carrying hereditary information and explained his discovery that certain characteristics were linked to the sex chromosomes. He revealed that different combinations of traits arise from changes occurring in the chromosomes during reproduction.
Thomas received the 1933 Nobel Prize for Medicine for his work in establishing the chromosomal theory of inheritance. His work with the Drosophila heralded a flurry of fundamental discoveries about inheritance.
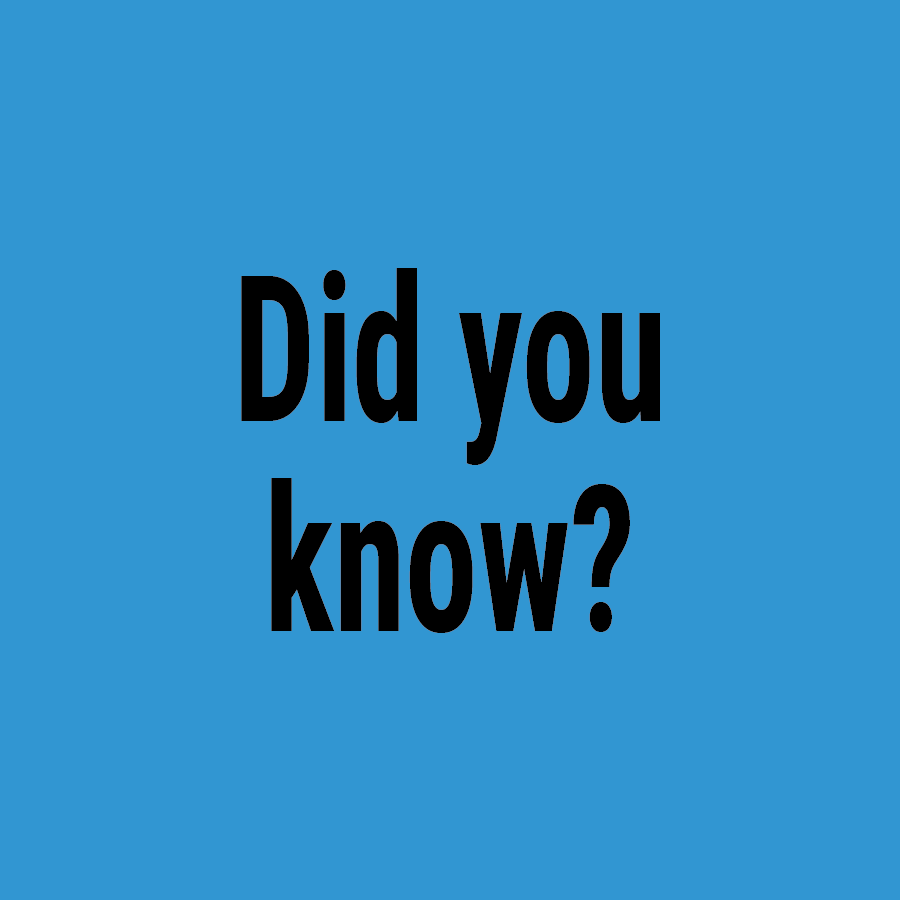
Thomas Hunt Morgan theorised that inheritance of the white-eye trait in fruit flies might have a basis in the chromosomes – and more specifically, the sex chromosomes.
The body structure and development of the fruit fly
Between the 1940s and early 1970s, Drosophila research continued to be productive.
Drosophila were bred where one or some genes were altered or removed completely. New techniques made it easier to handle flies in the laboratory. Important foundations were laid, yet the relationship between heredity and development went unresolved. There was a long period where it wasn’t clear whether the fly was going to yield anything of true significance.
Then, in the late 1970s and 1980s, genetics, embryology and molecular biology came together in a glorious union – and Drosophila research hit top gear. The new science of molecular biology brought with it the ability to manipulate DNA, and researchers could finally investigate the genes behind the mutated flies.
Some particularly influential genes were shown to cause strange transformations of the body structure. These are the genes on the ‘bithorax complex’, which control development on the rear and middle segments of a fly’s body, and the ‘antennapedia complex’ which control the head and front segments. See the below illustration as a guide.
Studies showed that the mutation of genes in the bithorax complex can lead to flies with two sets of wings or legs on their abdominal segments. Mutations in the antennapedia complex can lead to flies with legs where antennae should be.
The strangeness of these changes was a clue to the crucial role of the genes in the bithorax and antennapedia complexes – they turned out to be groups of master control genes that programme the final body structure of the fly.
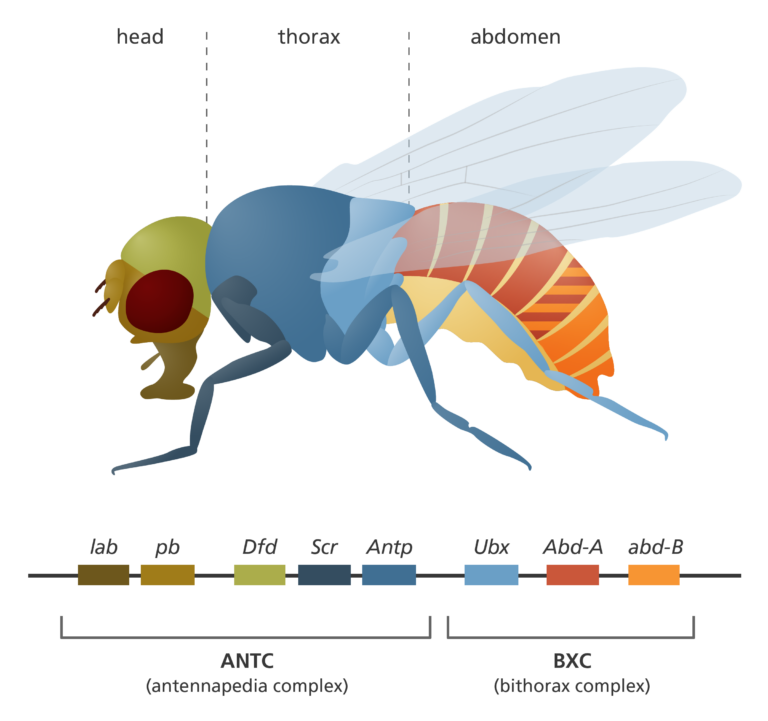
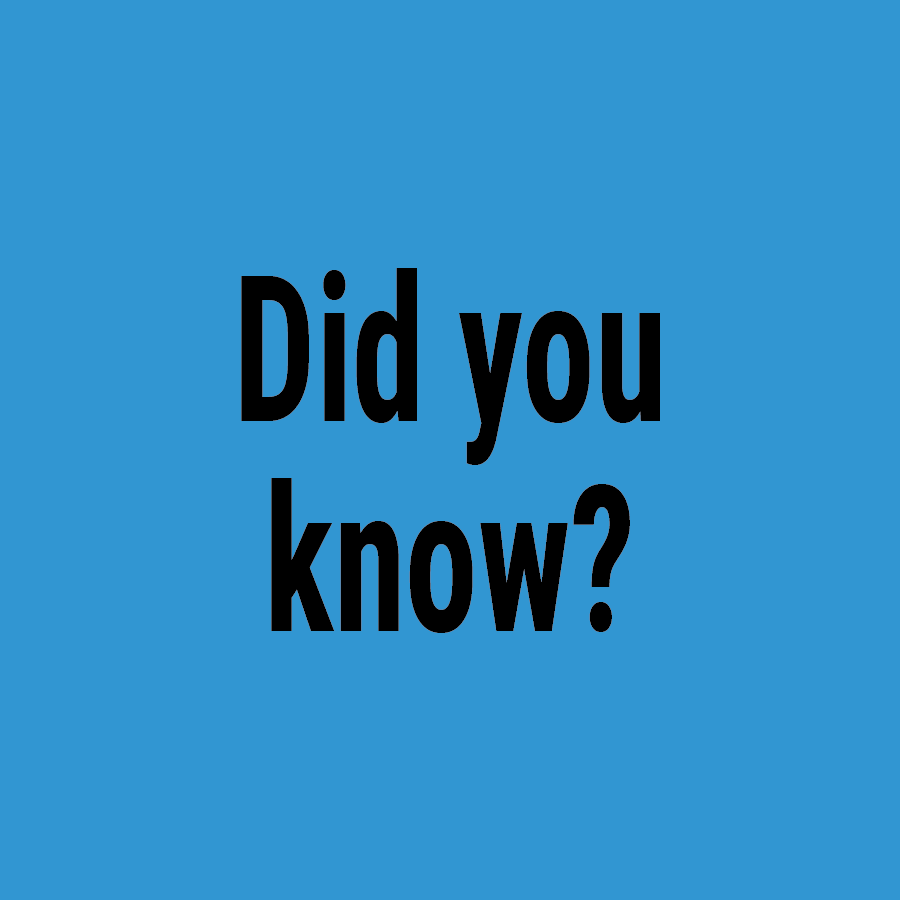
In the late 1970s and 1980s, Drosophila research hit top gear.
Discovering the genes involved in early body formation
Around this time, Christiane Nüsslein-Volhard and Eric Wieschaus began working together on the fruit fly in a small laboratory at the European Molecular Biology Laboratory in Heidelberg, Germany.
The duo examined thousands of fly embryos with mutated genes and disrupted development. As a result, they identified a collection of genes that drive the early development and the formation of body parts of the organism.
This new collection included:
- genes whose product is transferred from the mother to the embryo (called ‘maternal-effect’ genes). These are involved in specifying the major body axis (anterior-posterior and dorsal-ventral, or long-ways and width-ways).
- genes which are expressed by the embryo itself (called ‘zygotic segmentation’ genes). These help to define the different segments, such as the head, thorax and abdomen, of the developing embryo. These genes are broken down into ‘gap’, ‘pair-rule’ and ‘segment polarity’ genes.
Using the fruit fly to understand human genetics
The genome sequence of Drosophila has been available since 1999. From comparisons with the human genome, it’s thought that more than 75% of genes involved in human disease have counterparts in the fly. Scientists have used the fly to understand the way cells in our bodies communicate with each other, how our cells divide – and what happens when this process goes wrong, leading to the development of conditions like cancer.
Nearly a century on from Drosophila’s entrance on the world stage of biological research, this tiny fly has become one of the most well understood organisms there is.
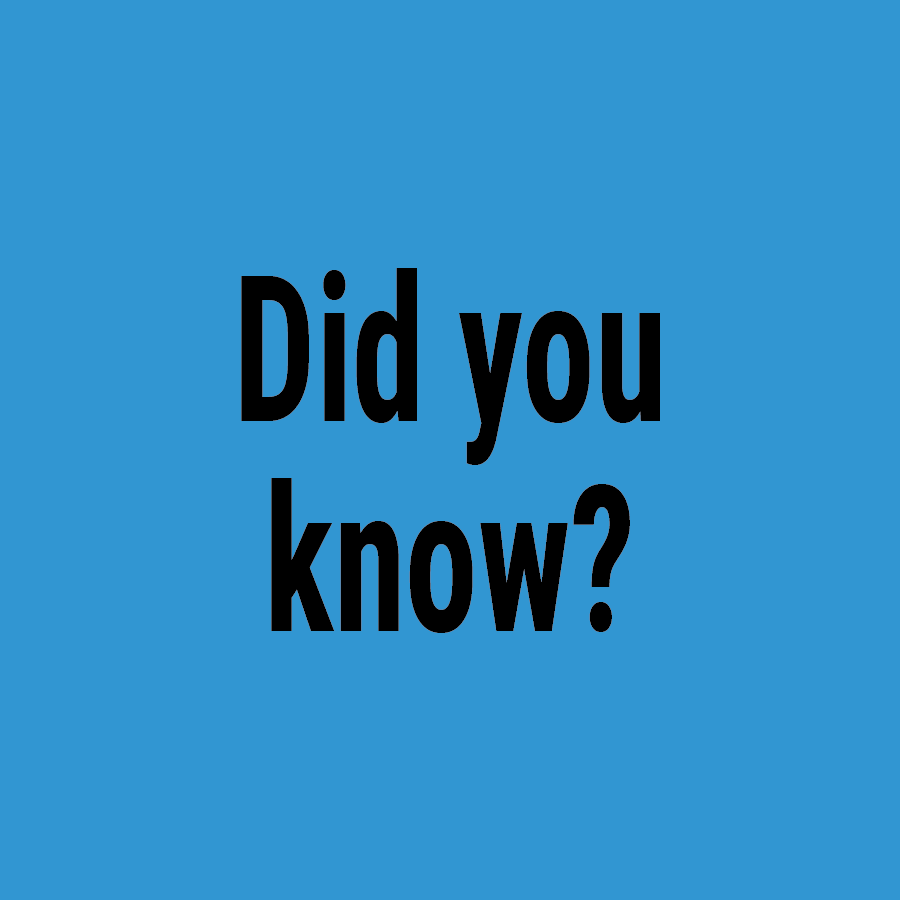